Institute of Advanced Study
Founded in 2006, the Institute of Advanced Study offers world-leading researchers a unique space for transformative reflection in and beyond individual disciplines.
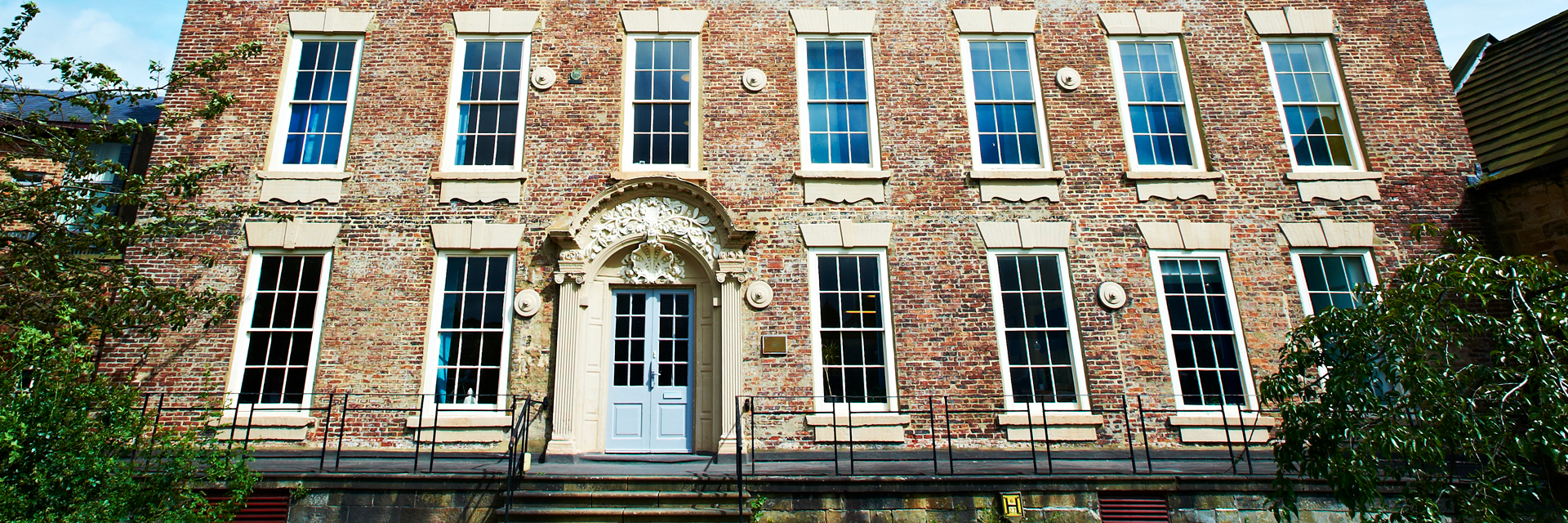
Welcome to the Institute of Advanced Study
Durham’s Institute of Advanced Study (IAS) is a home for growing big ideas. We support, promote and grow new and creative interdisciplinary ideas that transform our perspectives in challenging and provocative ways. We provide time, space, and resources to exchange and integrate ideas, knowledge and skills. We are a home to an inclusive, diverse and supportive community of scholars from Durham University and across the world.
Learn More About our Research
The Fellowship has not only been hugely productive, but it has had a profound effect on me. It has reminded me why I am an academic and has restored the love I have for the job.
Dr Peter Coe
University of Birmingham (March 2024)
Latest News
IAS Call for Research Development Projects 2025/26
From today we welcome applications for IAS Research Development Projects (RDPs) to run in academic year, 2025/26. Led by Durham academics, our RDPs are aimed to help and support the early-stage development of ambitious interdisciplinary research ideas and projects. Find out more about how to apply and the application deadline.
Research news
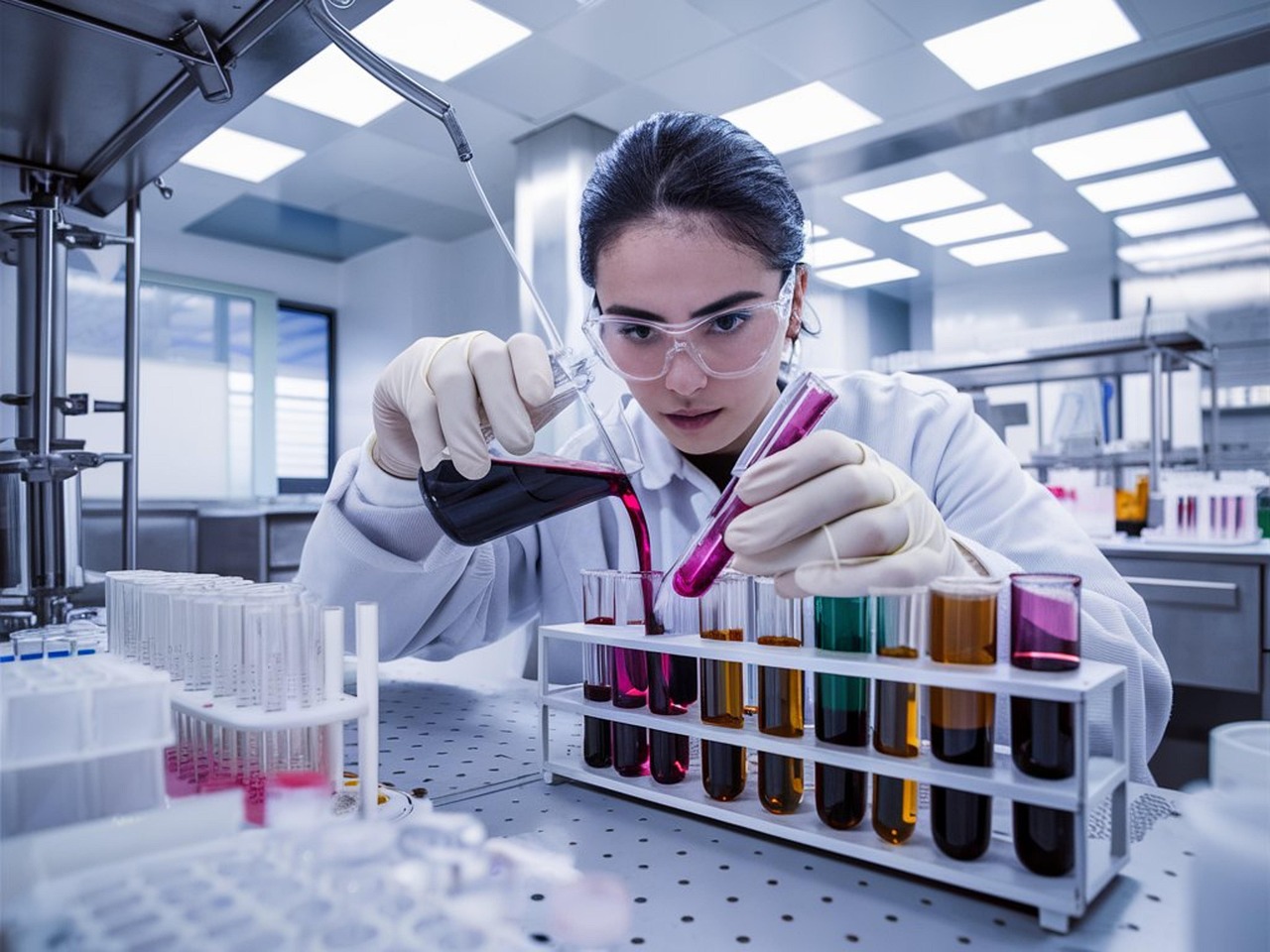
Welcome to IAS Epiphany term Fellows
We are delighted to have welcomed our second cohort of 2024/25 Fellows to the IAS to collaborate with colleagues on two major IAS projects: ‘Humans and Numbers’ and ‘The Many Facets of Social Inequality’, as well as other collaborations.
Research news
Matariki Institutes of Advanced Study support staff exchange between Matariki partners
Institutes of Advanced Study at four Matariki Universities, including the Durham IAS, are offering their communities as home for academic visitors travelling between Matariki partners.
Durham news
Publications, Videos and Social Media
Find out more about the Institute of Advanced Study including publications, details of former Fellows, and past projects.
Highlights
IAS Word Press
Keep up to date with the IAS newsletter, IAS videos, and historic IAS information
Publications
Explore some of the recent publications from IAS Fellows or from Durham colleagues supported by the IAS via funded projects and activities.
Social feeds
Get in touch
Enquiries about IAS should be sent via the email below.
Institute of Advanced Study
Durham University,
Cosin’s Hall,
Palace Green,
Durham,
DH1 3RL
Tel: +44 (0) 191 334 2589