Centre for Particle Theory
/prod01/prodbucket01/media/durham-university/departments-/physics/86219.jpg)
Centre for Particle Theory
The Centre for Particle Theory has earned an international reputation for its research. It unites the theoretical particle physics groups in the Physics and Maths Departments.
Strategic Importance and Added Value value
The Centre for Particle Theory is one of the largest particle theory groups within the UK (and larger than many departments in Durham). It covers nearly the whole spectrum of modern particle theory research.
More information
The CPT provides a critical mass of research excellence, and offers an in-depth training programme for the next generation of PhD students.
Our Pages
How to get to Durham
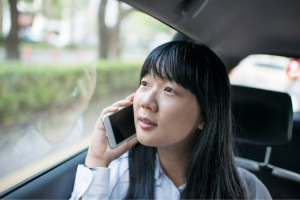
By car
Durham is about 30 min. by car south of Newcastle upon Tyne. Find the A1 and leave it at exit Durham.
.jpg)
By Bus
There are bus connections to Durham or Newcastle from most major cities in England.
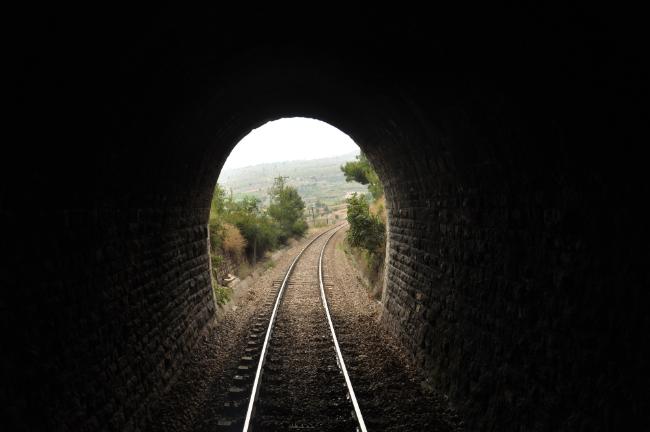
By Rail
The Intercity East-Coast line links Durham with London King's Cross and Edinburgh. Most Intercity trains stop in Durham. Otherwise you have to take a local train or taxi from Newcastle Central station. Timetable information is available from National Rail. Here is a link to help you finding your way through the London Underground.
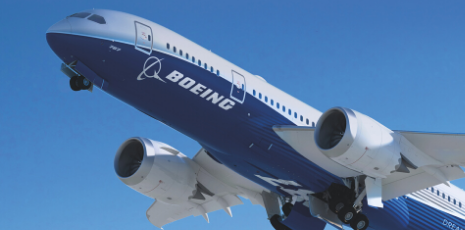
By Plane
Newcastle Airport has regular flights to and from Amsterdam, Brussels, Copenhagen, Dublin, Dusseldorf, Oslo, Paris and Stavanger as well as from London and other UK cities. To get to Durham take either the Metro which takes you to the Central station in Newcastle for about £5.70 then a train for about £6, or take a taxi to Durham which is about £60.
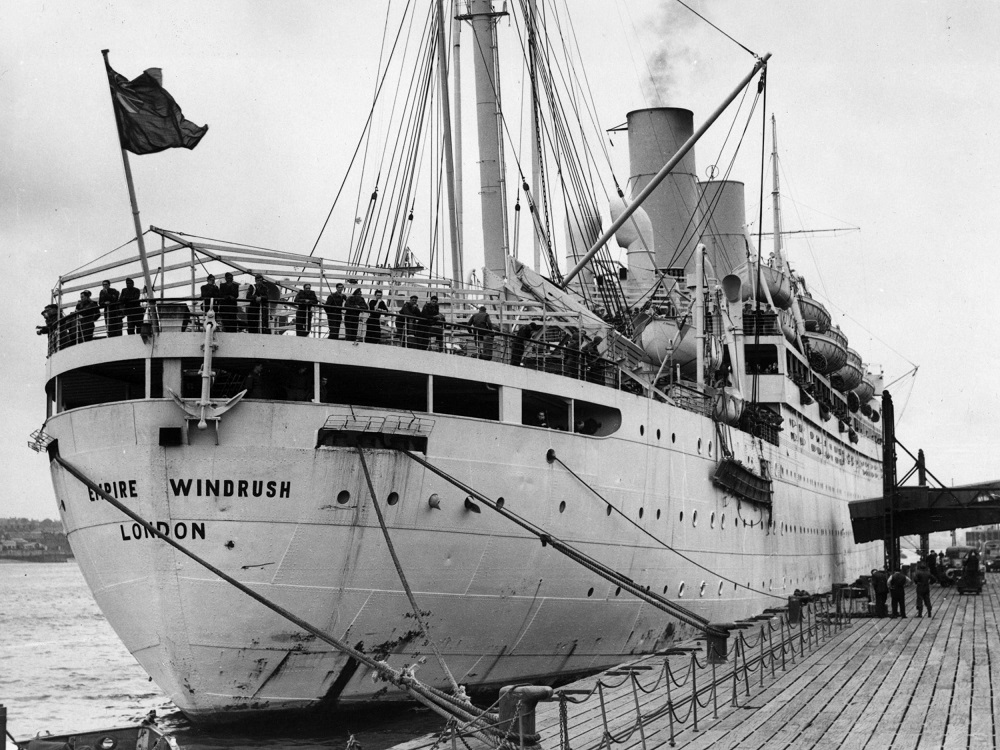
By Ship
There are ferry connection from Newcastle to Bergen, Esbjerg, Gothenburg, Hamburg, Oslo and Stavanger during summer. The crossing takes about 24hrs. Contact DFDS Scandinavian Seaways. The ferries arrive in Newcastle. There is a bus service to the central station. Trains to Durham run with a frequncy of more than 1 per hour.
Contact Us
Department of Mathematical Sciences and Department of Physics.
Durham University
Science Laboratories
South Rd
Durham
DH1 3LE
United Kingdom
0191 374 2349 (Maths)
0191 374 2167 (Physics)