Department of Chemistry
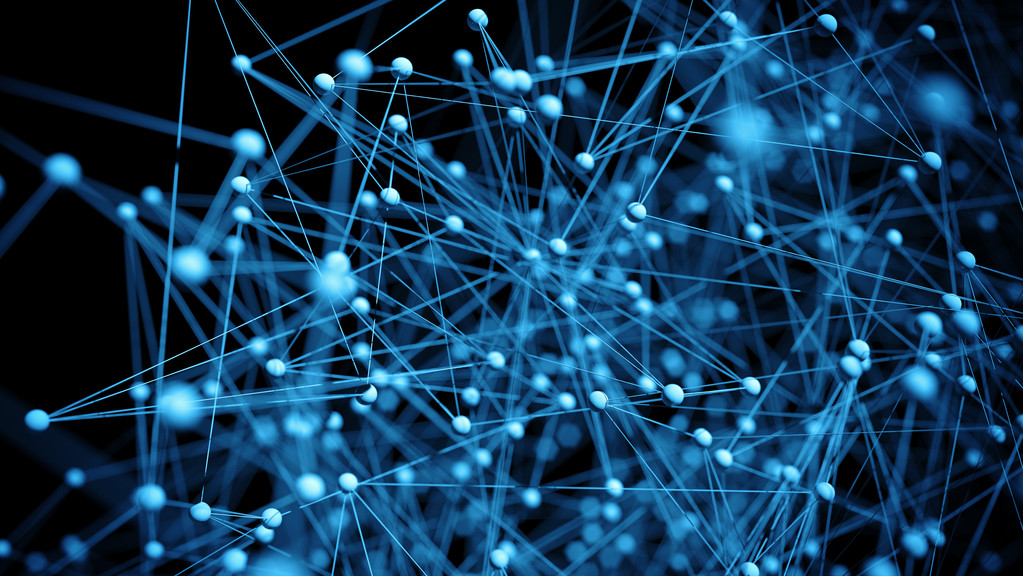
Welcome to the Department of Chemistry at Durham
We're proud to be a World Top 100 department with an extensive research base.
"It's incredible to watch our exceptional students develop as scientists and become highly engaged problem solvers ready to shape the future!"
Professor Chris Greenwell, Head of Department
What's new?
The global team on Neglected Tropical Diseases Wins the Durham Global SDG Award 2023
We are pleased to announce that the global consortium on Neglected Tropical Diseases led by Durham University won the inaugural Durham Global SDG award.
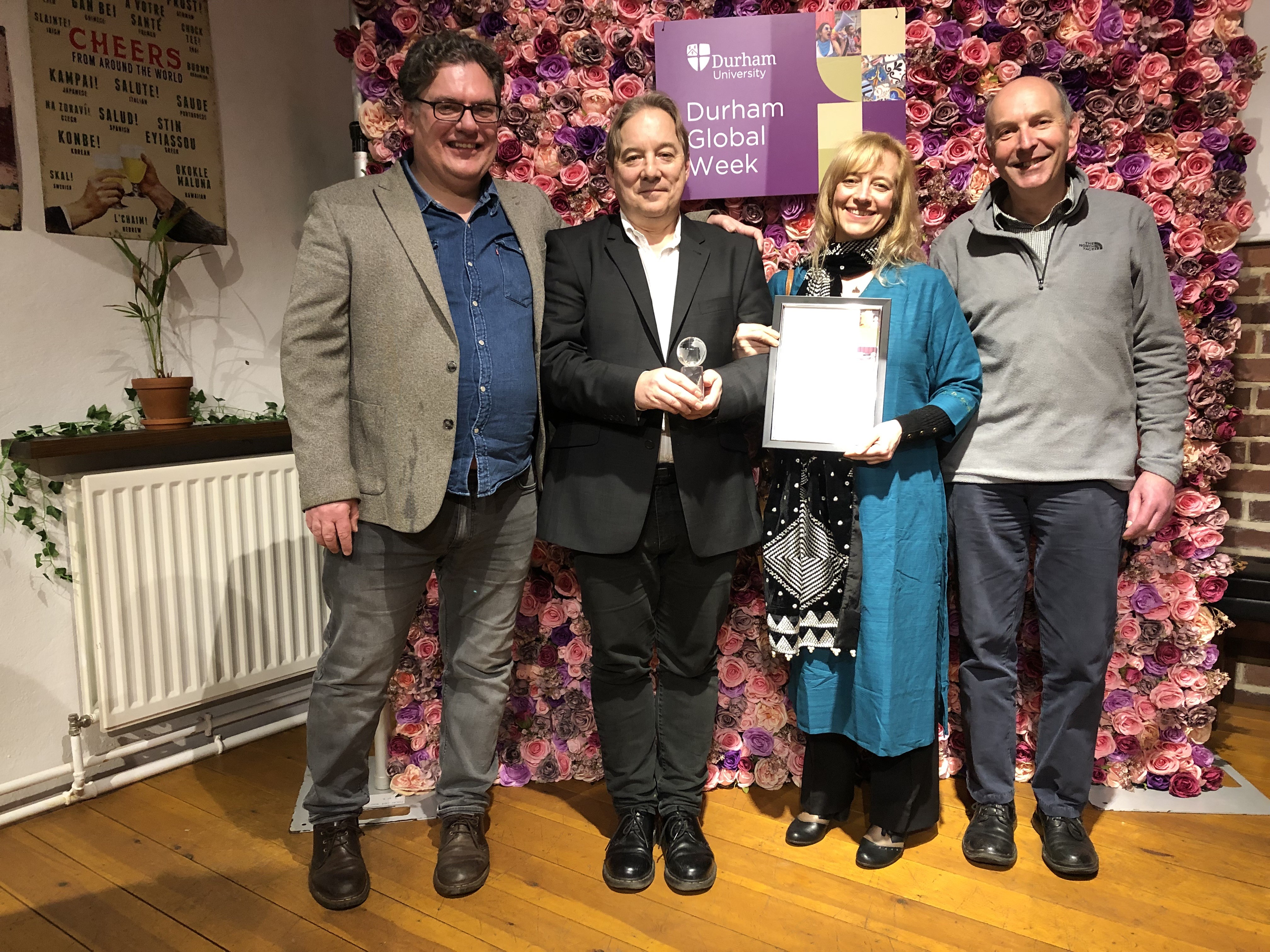
Professor Paul Denny and team announced as MRC Impact prize 2023 finalists in the Outstanding Team Impact category.
Ehmke Pohl and Paul Denny lead international workshop on neglected tropical diseases
Professor Jan Verlet wins prize from Royal Society of Chemistry
Respiratone shortlisted for Emerging Technologies Competition final
The 2023 Annual MoSMed CDT conference at the Helix in Newcastle
Chemistry PhD student shortlisted in prestigious awards
The global team on Neglected Tropical Diseases Wins the Durham Global SDG Award 2023
We are pleased to announce that the global consortium on Neglected Tropical Diseases led by Durham University won the inaugural Durham Global SDG award.
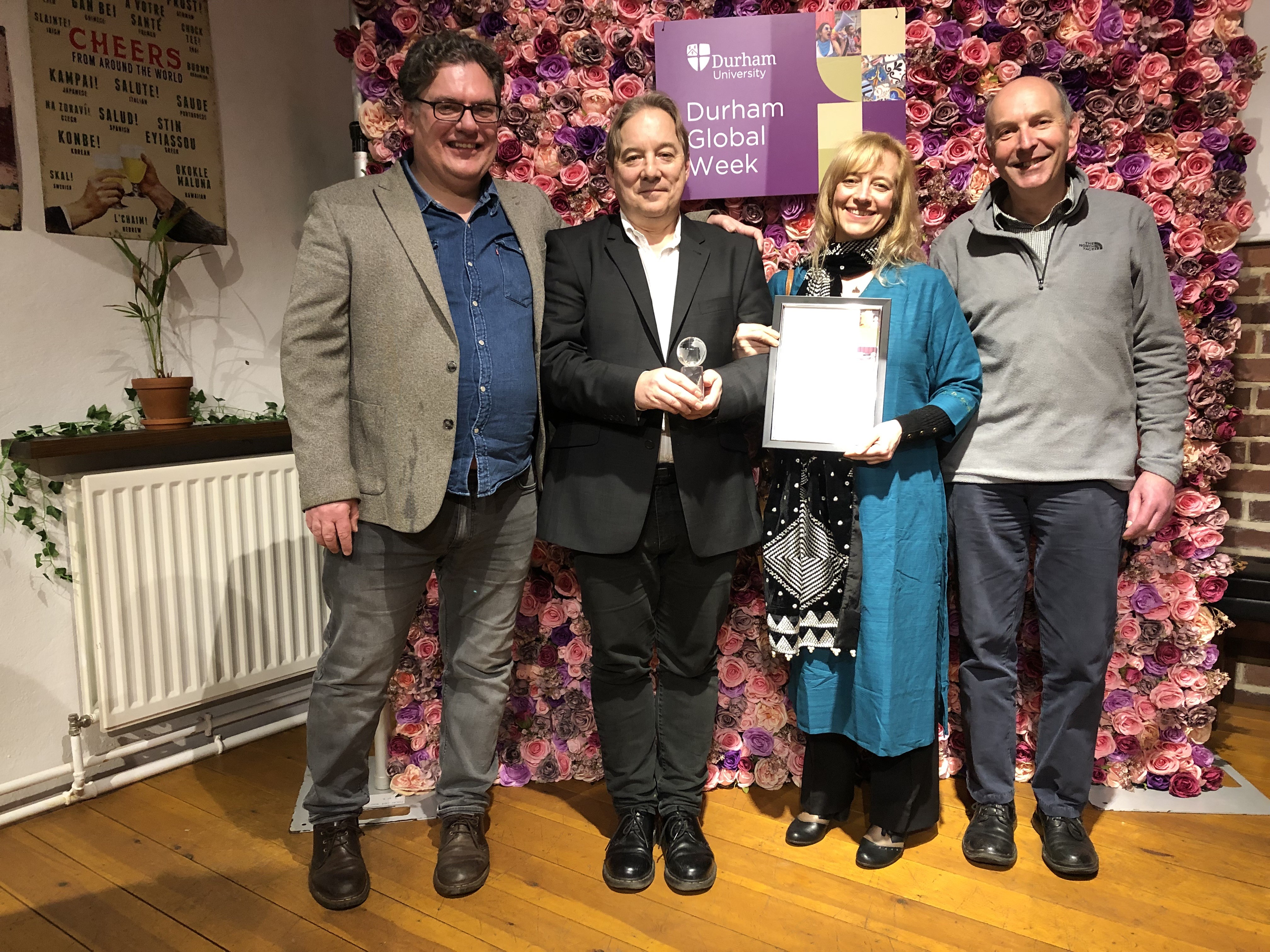
Professor Paul Denny and team announced as MRC Impact prize 2023 finalists in the Outstanding Team Impact category.
Celebrating Durham chemist’s place on prestigious development programme
Study with us
Undergraduate Study
We offer both BSc and MChem undergraduate courses, many accredited by the Royal Society of Chemistry.
Postgraduate Study
Discover our MSc and PhD research degrees at a world-leading university.
Our research
We are a vibrant, research-led department with an international reputation for cutting-edge science.
Diversity and Inclusion
Meet us and get involved
Our facilities and equipment
We provide services to our in-house researchers and students. We also work with many UK and overseas companies and other universities and research institutions.
Get in touch
Enquiries about Chemistry and our courses should be sent via the emails below:
Department of Chemistry
Durham University
Stockton Road
Durham
DH1 3LE
United Kingdom
Telephone (UK): +44 (0191) 334 2076
Telephone (International): +44 191 334 2000
Questions about studying here?
Check out our list of FAQs or submit an enquiry form.
Your Durham prospectus
Order your personalised prospectus and College guide here.